Understanding Qubits: Key Elements of Quantum Computing
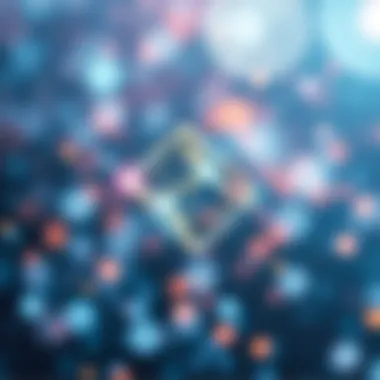
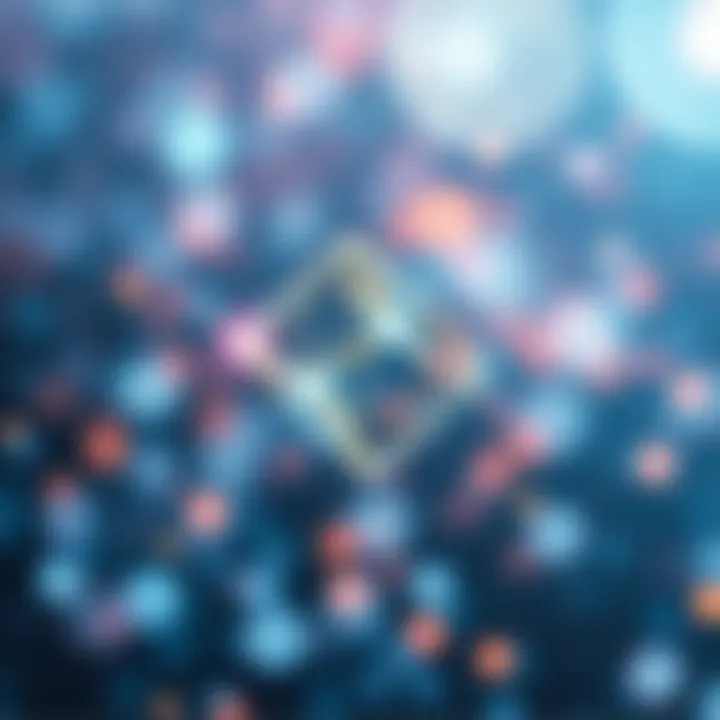
Intro
In the realm of computing, where traditional limits are constantly being pushed, a conversation has emerged about a peculiar yet fascinating concept: qubits. Unlike classical bits, which operate under a binary framework of 0s and 1s, qubits introduce a whole new ball game. Imagine this: a qubit can exist not just in a state of 0 or 1, but in both states simultaneously, thanks to the principles of quantum mechanics. This ability opens doors to computational capabilities that seem nothing short of magical.
Artificially constructing these qubits and harnessing their potential isnât just an academic exercise; it's a race against time in technology and innovation. Organizations across the globe are keenly interested in how quantum computing can revolutionize various sectors including cryptography, drug discovery, and artificial intelligence, essentially pushing whatâs feasible within our technological framework.
The quest for understanding qubits goes beyond mere definitions. Itâs about grasping the nuances that make them unique and understanding their broader implications. By appreciating the mechanics and behavioral traits of qubits, we can better envision a future where these quantum bits play a crucial role in solving complex problems that classical computers struggle with.
A curious mind could ponder: what makes qubits tick? How do they enable quantum algorithms to function? And just how far are we from fully realizing the potential that qubits herald? This article aims to unravel these questions and more, providing a comprehensive examination of how qubits stand as the building blocks of quantum computing.
The Concept of a Qubit
Understanding the concept of a qubit is essential for grasping the fundamental principles of quantum computing. Unlike classical bits, which serve as the building blocks of conventional computing and can either be in a state of 0 or 1, qubits offer a whole new dimension. They can exist simultaneously in multiple states, which is related to some of Quantum mechanics' most intriguing properties like superposition and entanglement. This incredible trait is not just a technical quirk; it vastly enhances computing power and versatility in ways hardly imaginable with traditional systems.
The qubit essentially embodies the strange and, at times, counterintuitive principles of quantum mechanics. It carries the promise of executing complex computations at speeds that would take classical bits eons to complete. Understanding these building blocks is crucial for anyone delving into the realms of quantum technology, be it educators conveying knowledge, investors assessing its potential, or developers crafting new algorithms.
"The power of qubits lies not simply in their ability to hold information, but in how that information can be manipulated to advance computation beyond current limitations."
In the sections that follow, we will establish a clearer definition of what a qubit is and highlight how it stands apart from classical bits. We will demystify the idea of quantum bits, increasing familiarity with the language and properties of this new paradigm. As we push on, keep in mind the implications qubits have for various sectors, from finance to healthcare, marking a radical shift in the potential of technology.
Defining the Qubit
A qubit, or quantum bit, serves as the elementary unit of quantum information. Analogous to a classical bit that can represent a 0 or a 1, qubits can achieve far more due to their ability to exist in a state of superposition, allowing them to represent both 0 and 1 simultaneously until measured. This duality lends itself to vast computational power, enabling quantum systems to perform calculations much quicker than their classical counterparts.
In practical terms, you can think of a qubit as a coin spinning in the air. While itâs spinning, it doesnât settle into heads or tails, representing a superposition of both states. Only when the coin lands do we observe a definitive outcome. This unique behavior is fundamental in quantum algorithms, where simultaneous processing of multiple possibilities is an invaluable asset.
Qubit vs. Classical Bit
When contrasting qubits with classical bits, the differences are stark and significant. Classical bits are limited to binary states, 0 or 1, but qubits blur these lines.
- Superposition: As mentioned, qubits can be in multiple states at once, exponentially increasing processing possibilities.
- Entanglement: Qubits can be entangled with one another, meaning the state of one qubit is directly related to the state of another qubit, regardless of the distance apart. This phenomenon plays a key role in quantum computing and provides advantages in data transfer and security.
The computational power of qubits, derived from their distinctive properties, allows quantum computers to solve complex problems like factorization and optimization tasks much more efficiently compared to traditional methods. This capability heralds changes across industries and paves the road toward advances in cryptography, drug discovery, and artificial intelligence.
In summary, recognizing the unique characteristics of qubits compared to classical bits lays the groundwork for understanding their pivotal role in the ever-evolving landscape of quantum computing. The journey continues as we dive deeper into the mathematical frameworks that define how qubits function in quantum systems.
The Mathematical Framework
The mathematical framework is crucial to comprehending the intricate world of qubits. It offers the theoretical foundations that allow us to grasp how qubits function and behave in contrast to their classical counterparts. The beauty of quantum mechanics lies in its ability to quantify the seemingly impossible phenomena that can be observed in a quantum system. By understanding the mathematics behind qubits, one becomes equipped to appreciate the paradigm shift they bring to the realm of computation.
The framework is not just a collection of equations and symbols; it opens the door to understanding phenomena such as superposition and entanglement. These concepts are not only fascinating but also pivotal in unlocking quantum technology's unparalleled computational power. In terms of application, the mathematical aspects directly contribute to the development of quantum algorithms, which may offer elegant solutions to problems that have historically been regarded as insurmountable.
Quantum Superposition
In the quantum world, superposition embodies the idea that a qubit can exist in multiple states simultaneously, not merely one or zero as in classical bits. This characteristic is akin to flipping a coin: while it's in mid-air, it can be viewed as both heads and tails until it lands. This idea is famously illustrated by Schrödinger's cat thought experiment, wherein the cat can be both alive and dead at the same time until observed.
Mathematically, this is represented using a combination of vectors, most commonly in a two-dimensional space known as Hilbert space. A qubit is mathematically expressed as:
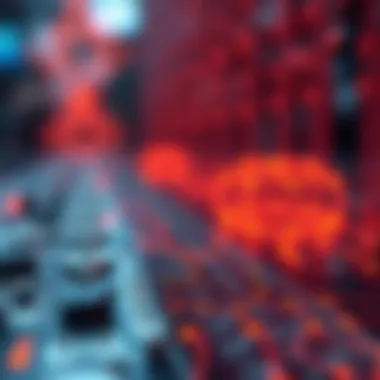
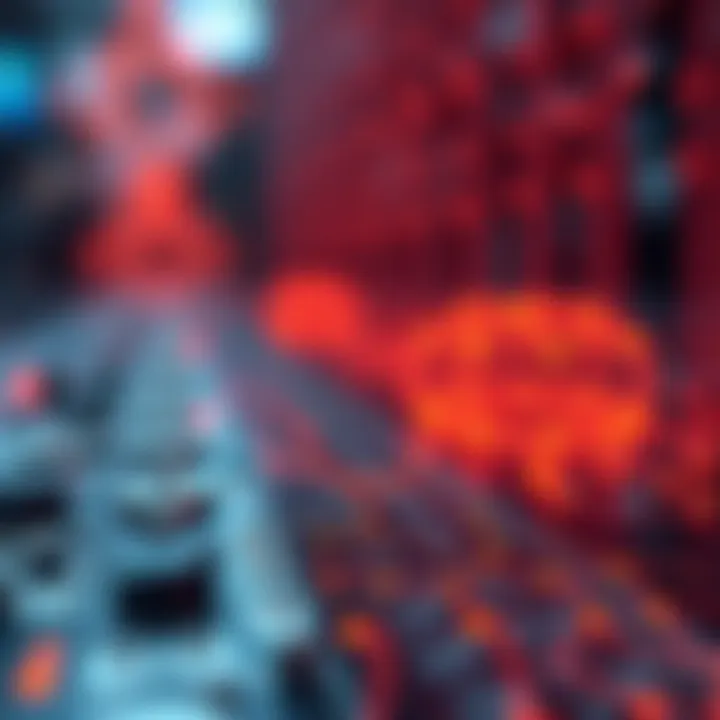
$$| extq \rangle = \alpha | 0 \rangle + \beta | 1 \rangle$$
Here, ( |0â© ) and ( |1â© ) denote the state of the qubit, while ( \alpha ) and ( \beta ) are complex coefficients that describe the probability amplitudes associated with these states. The probabilities of measuring the qubit in either state are given by the squares of the absolute values of these coefficients:
- Probability of obtaining ( |0â© ): ( |\alpha|^2 )
- Probability of obtaining ( |1â© ): ( |\beta|^2 )
This framework, albeit non-intuitive, significantly enhances computing capabilities. A set of qubits in superposition can process information in a way that classical bits never could, allowing quantum computers to tackle complex problems much more efficiently.
Quantum Entanglement
Entanglement takes the peculiarity of quantum mechanics a step further. When two qubits become entangled, the state of one qubit is directly linked to the state of the other, regardless of the distance between them. This means altering one qubit will instantaneously affect its entangled partner. Einstein famously referred to this phenomenon as 'spooky action at a distance,' emphasizing its counterintuitive nature.
Mathematically, if we denote two qubits in a combined state, we can express this entanglement with:
This represents a state where if the first qubit is measured to be 0, the second qubit will also be found to be 0, and the same goes for 1. The implications of such relationships are profound; entangled qubits can perform computations that leverage this interdependence, lending them an extraordinary advantage in certain computational contexts.
To sum up, both superposition and entanglement are critical components of the quantum mathematical framework. They enable the unique capabilities of quantum computing, marking a tangible shift in how we approach computational problems. Understanding these concepts not only aids in appreciating quantum mechanics but also serves as a stepping stone for those aiming to navigate the burgeoning field of quantum technology.
"In quantum mechanics, the principle of superposition and the phenomenon of entanglement are foundational, illuminating the fascinating interplay between nature's most basic laws and the computational power we strive to harness."
For further insights, you can explore resources like Wikipedia, Britannica and discussions on Reddit related to these concepts.
Physical Realizations of Qubits
In the ever-evolving world of quantum computing, understanding qubits requires more than just grasping their theoretical underpinnings. The practical applications and physical realizations of qubits are crucial for harnessing their potential in real-world scenarios. Different methods of creating and manipulating qubits can lead to varied implications for performance, scalability, and error rates.
When we talk about physical realizations, weâre essentially looking at how qubits are constructed and how they operate within a quantum computing system. This not only helps in advancing quantum technologies but also sheds light on the feasibility of deploying such systems across different industries.
Trapped Ions
Trapped ions are one of the more established methods of realizing qubits. In this approach, individual ions are confined using electromagnetic fields, allowing them to serve as quantum bits. Hereâs a look at some of the significant features of trapped ions:
- High Coherence Times: Trapped ions exhibit longer coherence times compared to other qubit types, which is crucial for maintaining quantum states during computations.
- Addressability: Each ion can be precisely addressed using lasers, enabling complex quantum operations to be performed efficiently.
- Scalability Challenges: While highly accurate, scaling ion traps to accommodate a large number of qubits can be difficult due to physical space and cooling requirements.
"Trapped ions represent a clear pathway towards achieving quantum supremacy, demonstrating unparalleled precision in qubit manipulation."
Despite their challenges, trapped ions have been successful in quantum research labs, pushing the boundaries of what is achievable in quantum systems.
Superconducting Circuits
Next up are superconducting circuits, a robust approach that has gained significant attention. These qubits leverage the properties of superconducting materials to achieve quantum behaviors at macroscopic scales. Here are some relevant points:
- Versatile Design: Superconducting qubits can be designed in various configurations, such as transmons and fluxonium, each offering unique advantages depending on the application.
- Fast Gate Operations: They enable rapid switching times, making them ideal for executing quantum algorithms efficiently.
- Decoherence Issues: Superconducting qubits face challenges linked to environmental noise, leading to decoherence, although ongoing research aims to mitigate these effects through improved materials and technologies.
The versatility and relatively straightforward fabrication of superconducting circuits place them at the forefront of quantum computing initiatives, being utilized by companies like Google and IBM.
Topological Qubits
Lastly, we have topological qubits, which draw inspiration from topology, a branch of mathematics. Unlike other qubit forms, topological qubits aim to encode information in a way that is inherently robust against local disturbances.
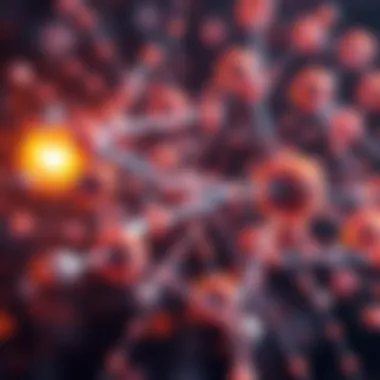
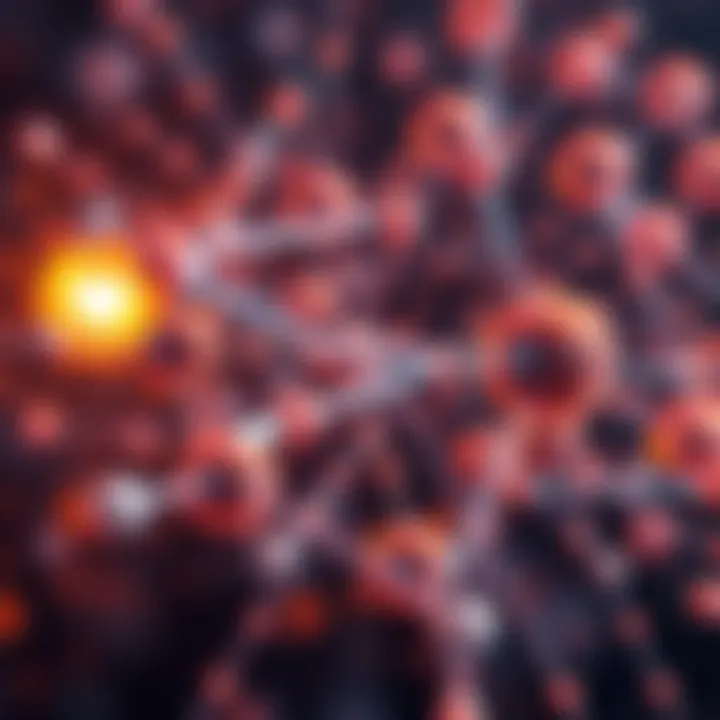
- Error Resilience: Their structure makes them less vulnerable to local noise, which is a significant advantage in maintaining coherence during computations.
- Complexity of Creation: The physical realization of topological qubits presents challenges; for instance, creating and maintaining Majorana fermions necessary for these qubits involves intricate experimental setups.
- Potential Applications: If mastered, topological qubits could revolutionize quantum computing by providing a stable qubit solution usable in various practical applications.
In summary, the assortment of physical realizations for qubits reveals a landscape filled with possibilities, challenges, and a wealth of ongoing research. Each approach contributes to a broader understanding of how to harness quantum principles effectively to support advancements across technology sectors. For more insights, check out resources like Wikipedia or Britannica.
With practical realizations ranging from trapped ions to superconducting circuits and the promising future of topological qubits, the journey towards effective quantum computing continues.
The Role of Qubits in Quantum Computing
When we look closely at the landscape of quantum computing, it's clear that qubits sit at the epicenter of this revolution. Unlike their classical counterparts, qubits aren't just simple on-or-off switches. They introduce a realm of possibilities that traditional computing fundamentally cannot explore. Their role is not only critical but transformative.
Quantum Algorithms
At the heart of quantum computing's promise lies the concept of quantum algorithms. These are designed specifically to utilize the peculiar properties of qubits, such as superposition and entanglement. For example, Shor's algorithm, which factors large integers in polynomial time, directly challenges the fundamental grunt work of current classical algorithms.
Utilizing qubits allows for parallel processing, meaning that multiple calculations can occur simultaneously. This effect can drastically reduce computation times for problems that are cumbersome, if not outright infeasible, using traditional methods. When we think of factors such as cryptography, optimization problems, and simulations of quantum systems, the benefits of quantum algorithms start to come into sharper focus.
"Quantum computers solve problems in seconds that would take classical computers millions of years." - Unknown
The power of these algorithms is not solely rooted in their speed; they also offer solutions that were previously out of reach. Creating new drugs, understanding complex materials, and decoding intricate biological processes are just some applications where quantum algorithms shine. They usher in a new era where innovation can thrive, making the development of efficient quantum algorithms pivotal.
Speedup Over Classical Computing
The allure of qubits isn't just their ability to represent multiple states simultaneously; it's how they translate this capability into practical speed advantages over classical computing systems. Take, for example, Grover's search algorithm, a quantum algorithm that demonstrates quadratic speedup. While classical algorithms may require checking every possible answer in a list, Grover's approach allows for significantly fewer checks, leveraging the power of superposition.
The implications are profound. For instance, what might take a classical computer hours could be achieved by a quantum computer in mere minutes. This speedup leads to more than just efficiency; it can alter the course of industries. We can conceptualize this speedup through the lens of industries heavily reliant on data analysis such as finance, healthcare, and logistics. Tasks involving vast databases can be executed in record time, while decision-making processes become faster and more informed.
To illustrate, a commonly cited example is the optimization of delivery routes for logistics firms. With the intense complexity involved in assessing thousands of potential routes, classical computing can be bogged down. But a quantum computer harnessing qubits could sift through these options much faster, providing optimized routes in a fraction of the time.
It's important to note, however, that not all problems naturally fit this new model. The growth of quantum computing has made it crucial to identify which problems align with the strengths of qubits. This assessment will guide future research, algorithm development, and practical applications.
In summary, qubits play a pivotal role in the evolution of computing technology. As we step into an age where their potential becomes more fully realized, it is essential to grasp their capabilities and limitations. Understanding this will not only help industry leaders harness the power of quantum algorithms but will also shine light on the impending changes across various fields.
Challenges in Qubit Technology
As we plunge deeper into the world of quantum computing, it becomes crystal clear that while qubits are the cornerstone of this new technological frontier, they come with their share of hurdles. Understanding these challenges is crucial, as they not only shape the development of quantum systems but also influence their commercial viability and operational efficiency. The main players in this arenaâtraders, investors, educators, analysts, and developersâmust navigate these complexities to fully harness the power of qubits.
Decoherence
Decoherence, the bane of many quantum systems, refers to the loss of quantum states due to interactions with the environment. In simpler terms, it's like trying to keep a delicate soufflĂ© from collapsingâany slight perturbation can spoil the whole thing. When qubits interact with external noise or disturbances, they tend to lose their quantum properties, transforming into classical bits. This process happens extremely quickly and often at a rate faster than we can develop solutions.
Given that decoherence poses significant risks in maintaining the integrity of qubit information, researchers are engaged in a myriad of strategies to mitigate its effects. Some of these include:
- Isolated Qubit Systems: By physically separating qubits from their surroundings, it's possible to reduce interaction rates that could induce decoherence.
- Material Science Innovations: High-quality materials can significantly reduce the noise that contributes to decoherence. For instance, superconducting materials are often explored for their lower noise profiles.
- Environmental Control: Advanced temperature regulation and electromagnetic shielding may help in maintaining a coherent state for longer durations.
The battle against decoherence is ongoing. By addressing this issue effectively, we can enhance the lifespan and usability of qubits, moving us closer to their potential real-world applications.
Error Correction
Even if we succeed in extending coherence times, the reality is that errors will inevitably arise in quantum computations due to imperfections in qubit design or external disruptions. Error correction thus becomes a fundamental part of working with qubits, akin to having a backup plan when navigating through uncharted territory.
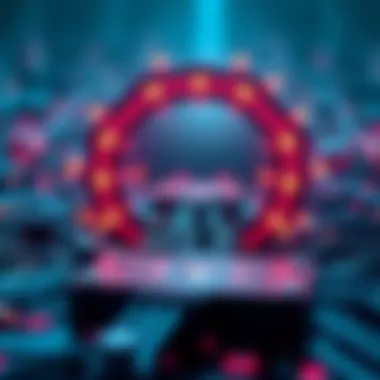
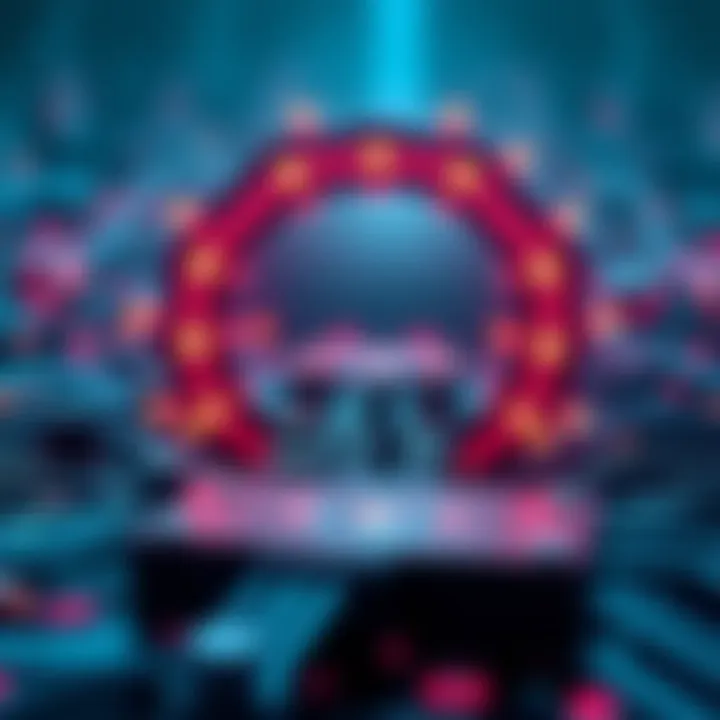
Quantum error correction (QEC) is not as straightforward as classical error correction methods. In classical systems, errors can often be detected and corrected by simply checking bits against their expected values. However, in the quantum realm, due to the no-cloning theorem and the fragile nature of quantum states, simply measuring a qubit can disrupt the state.
Some strategies in quantum error correction include:
- Redundant Qubit Encoding: Qudits, higher-dimensional analogs to qubits, can store more information and help mitigate errors. Quantum information can be preserved across several qubits via careful encoding schemes.
- Threshold Theorems: These theorems provide guidelines on how much error a quantum system can tolerate before it becomes unusable. This concept is pivotal for building scalable quantum systems.
- Topologically Protected Qubits: These qubits are designed to resist decoherence and other errors by relying on the topology of the materials themselves, significantly reducing noise and making them less susceptible to errors.
In summary, tackling the dual challenges of decoherence and error correction is essential for the survival and advancement of qubit technology. The outcomes of these efforts will ultimately shape the landscape of quantum computing and determine the pace of innovation in the field. Investing in research to overcome these hurdles could unlock unimagined potentials in numerous sectors, making it a matter of keen interest for all involved stakeholders.
"The path to practical quantum computing is paved with challenges, but with each step forward, we inch closer to unlocking its transformative potential."
For additional insights on quantum technology, you may refer to resources like Wikipedia or Britannica. Understanding these challenges is not just an academic exercise; it's a critical step towards tangible advancements.
The Future of Qubits and Quantum Computing
The trajectory of quantum computing is largely dependent on the advancement of qubit technology. As we stand at the precipice of a computing revolution, understanding the future of qubits becomes imperative. The implications of the ongoing research and technological developments can significantly alter various industries, enabling solutions to problems that were previously thought insurmountable.
Imagine a world where calculations that take supercomputers years might be crunched within seconds thanks to the capabilities of quantum computing. The need for more efficient computation arises from our ever-increasing data demands, and qubits are set to play a pivotal role in meeting this need through enhanced computation capabilities. Let's dive deeper into what this future involves.
Emerging Research Directions
Various innovative paths are being explored as researchers strive to bolster qubit performance, reliability, and scalability. Some of the prominent research avenues are:
- Quantum Error Correction: This is a hot topic as it aims to mitigate the effects of decoherence and operational errors in qubit systems. Improving methods to combat these issues is crucial for practical quantum computation.
- Qubit Interfacing: Scientists are looking at how to enhance communication between qubits while ensuring their stability. This could improve the integration of qubits in larger systems, making quantum processors more efficient.
- Hybrid Quantum-Classical Systems: By integrating classical systems with quantum ones, researchers explore how they can work together. This blends the best of both worlds, leveraging existing technology while pushing forward innovations.
"The future of quantum computing hinges on mastering qubits â their control, coherence, and the ability to harness entanglement on a larger scale."
- Topological Qubits: Pioneers are investigating these exotic particles, which theoretically have built-in error resistance. They hold the promise for stable, long-lived qubits ideal for computation.
Continued funding and collaboration across academia and industry will be key to pushing these frontiers further. As every scientist knows, each step forward can lead to unforeseen opportunities and pathways.
Potential Applications
It's one thing to envision a better future for qubits; itâs another to consider their practical applications once developed adequately. Here are some exciting prospects:
- Cryptography: Quantum computing is poised to redefine security protocols. Algorithms like Shorâs can effectively crack existing encryption methods, pushing cryptographers to develop quantum-resistant systems.
- Drug Discovery: The healthcare sector stands to benefit immensely as quantum computing enables complex simulations of molecular interactions, potentially accelerating the discovery of new drugs.
- Financial Modeling: In finance, the vast amount of data processed can be optimized through quantum algorithms, allowing for better predictions of market trends and risk assessments than classical computing can achieve.
- Artificial Intelligence: AI techniques, especially in machine learning, can be enhanced significantly as quantum systems can explore vast datasets more effectively than their classical counterparts.
- Climate Modeling: These advanced computing solutions can also offer robust models to tackle climate change, assisting scientists in simulating and forecasting complex climate phenomena.
The prospect of leveraging qubits for real-world applications shows great promise, yet these technologies must first overcome numerous barriers including practical deployment and cost-effectiveness.
In sum, the future of qubits in quantum computing not only encompasses groundbreaking research but also influences a myriad of fields that touch our everyday lives. The road ahead seems filled with innovation, and as we navigate this technological landscape, the effective integration of qubits into various applications could change how we perceive computing.
Culmination
As we reach the end of our exploration into the world of qubits, itâs essential to emphasize their critical role in the advancement of quantum computing. The evolution of computing from classical bits to qubits marks a significant watershed moment. Unlike classical bits, which are either a 0 or a 1, qubits can exist in multiple states at once, thanks to the principles of superposition and entanglement. This capacity underpins their ability to perform complex calculations at remarkable speeds, enticing traders and investors to consider new opportunities in quantum-enhanced technologies.
In this article, we examined several facets of qubits:
- Definition and Characteristics: Understanding what makes qubits unique was our starting point. Their ability to represent both 0 and 1 simultaneously enables a level of processing power unattainable by classical computing methods.
- Mathematical Framework: We dove into the intricate math behind quantum states and how superposition and entanglement can be harnessed to execute quantum algorithms more efficiently.
- Physical Realizations: By discussing trapped ions, superconducting circuits, and topological qubits, we also showcased the different ways researchers are attempting to stabilize and scale qubit systems.
- Role in Quantum Computing: Analyzing how qubits facilitate advancements in quantum algorithms provides insight into their profound implications.
- Challenges: We highlighted the hurdles that remainâsuch as decoherence and error correction, which are crucial challenges that need addressing for real-world application.
- Future Directions: Lastly, we speculated on the trajectory of quantum computing, noting emerging research that may redefine industries, from finance to drug discovery.
"The shift to quantum computing represents not just an enhancement but a transformation in how we process information, making once-daunting computational tasks possible."
Recap of Qubit Importance
In summary, qubits are not just a theoretical concept but the driving force behind future technologies. They hold the potential to streamline processes, enhance algorithms, and possibly solve problems currently deemed intractable. For traders and developers, understanding qubits isnât merely about quantum theory; itâs about recognizing the next wave of innovation and its applications across domains.
As industries begin to harness the power of quantum computing, the demand for knowledge about qubits will continue to growâmaking it imperative for those involved to stay informed and adapt. Ultimately, the transformational capabilities of qubits could redefine our approach to computing and data processing, opening up a realm of possibilities previously thought to be the stuff of science fiction.